Surgeon Phoenix works in a wide variety of workplace settings. Their main responsibilities include preoperative diagnosis of patients, performing surgery and postoperative treatment, and supporting other surgeons through administration duties such as arranging rotas and paperwork.
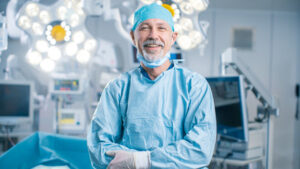
People in this career tend to enjoy challenging work and feel a sense of achievement. They also show a preference for independent and autonomous work.
The educational requirements for a surgeon are incredibly demanding. Those pursuing this career will need no less than 13 years of education and training before they can begin practicing in the medical profession. It takes an in-depth understanding of the human body to perform surgical procedures that are meant to investigate disease, repair damaged tissues or replace a lost organ. The field of surgery requires exceptional manual dexterity, so many doctors with this specialty will have additional certification in areas such as occupational therapy and physical therapy to prepare them for their work.
The first step is earning a degree from an accredited college or university. A student should select a major in a subject related to medicine and take all prerequisite courses required for entry into medical school. Future doctors will need to pass the Medical College Admission Test (MCAT) during undergraduate school, and then complete a four-year program that teaches them basic medical skills and introduces them to various specialties through clinical rotations.
After graduating from medical school, aspiring surgeons will complete a residency program in their chosen specialty. This program can last between five and seven years and involves hands-on training under the supervision of a senior surgeon. During this time, the aspiring surgeon will learn to work with patients and help them overcome various health issues and injuries. Some surgeons will opt to add further specialized training by participating in a fellowship, which is a one- to three-year program that allows them to focus on an area of the medical field they are passionate about.
Surgical Residencies
Surgical residencies are a time of a resident’s life when he or she learns to perform and think about medical problems in a unique way. They typically involve long hours, a high degree of responsibility and intense educational experience. During the residency, students learn to evaluate and plan for the treatment of patients; attend rounds, outpatient clinics and conferences; and assist in the operating room. The residents work as members of the assigned surgical care team, under the supervision of an attending surgeon.
In general, a surgery residency involves three years of clinical patient care and two or more years of research. In addition to providing state-of-the-art and compassionate patient care, a good surgery program focuses on developing residents as well-rounded individuals who will contribute to the preservation of surgical knowledge through education. The clinical training includes exposure to general, hepatobiliary and vascular surgery, as well as a variety of pediatric, endocrine and colorectal surgeries. Some programs also offer a Global Surgery rotation that provides the opportunity for residents to take part in humanitarian-oriented rotations in underdeveloped or underserved countries around the world.
Some of the top surgery residencies are affiliated with major universities, and many have a strong reputation in both education and clinical care. They have highly skilled teaching faculty and state-of-the-art facilities, including simulation laboratories for surgical skills. Residents are given ample opportunities to participate in basic science, translational and clinical research and are encouraged to present their work at local and national venues.
Students interested in a surgical residency should try to get a feel for a particular program before applying. A subinternship can help them determine whether the institution and the program are a good fit, and it may give them a better chance of being selected for a categorical position. If possible, it is also a good idea to apply for a preliminary position rather than going unmatched. This way, they can obtain an excellent residency even if they do not secure a categorical spot. This is because if a program experiences high attrition from its categorical positions, the openings can often be filled by preliminary residents.
Working Conditions
In general, physicians and surgeons work in clean, well-lit operating rooms with sterile equipment. They are usually assisted by nurses, medical assistants, laboratory technicians, bookkeepers and receptionists. Surgeons may choose to practice solo, or to join a group practice, a health care organization or hospital. Group practices provide backup coverage, a schedule for meetings with patients and the opportunity to share knowledge.
Surgeons are at risk for occupational hazards that can affect their physical health, including back and neck pain, as well as other musculoskeletal problems. They also are at risk for mental health issues such as depression and burnout, which can result from high levels of stress.
Performing surgery makes up the majority of a surgeon’s workday, but it is not the only important task. Surgeons also undertake ward rounds, surgical meetings and consultations and report-writing. In one study, the amount of time that surgeons spent on tasks that were not related to patient care was a significant predictor of their daily job satisfaction (Szeto et al. 2009).
A number of surgeons have reported a range of physical health complaints, such as hip and knee discomfort, forearm, wrist/hand or neck pain and stiffness and shoulder complaints. These are a consequence of awkward body postures and repetitive movement, prolonged static positions and working conditions that can lead to muscle tension and fatigue.
These work-related musculoskeletal complaints are a major contributor to surgeons’ absenteeism, which is greater than that of other professions in the same industry. These symptoms also affect the quality of their life and can cause them to become distracted from their work duties and irritable and aggressive towards colleagues and patients.
In a recent study, a representative sample of a cohort of hospital surgeons and physician-hospitalists completed a questionnaire that asked about their daily job satisfaction, work ability, work-related physical health complaints, and perceived level of control over their workload. The results indicated that the surgeons’ favorite task was performing surgery; this was followed by teaching and continuous education. Administrative tasks, on the other hand, were the least attractive. Moreover, the results of the study showed that the surgeons’ perception of the legitimacy of their administrative work influenced their daily job satisfaction to a significant degree.
Job Duties
Surgeons are medical professionals who focus on diagnosing and treating illnesses that may call for surgery or other physical alterations to the body. Their job involves extensive education and training, including four years of undergraduate study, four years of medical school, and 5-7 years of residency in their chosen specialties. They often work long hours and have to be on call at all times, which can impact their work-life balance. However, a surgeon’s career provides intellectual challenge and the opportunity to make a real difference in people’s lives.
A surgeon’s job duties include examining patients, obtaining medical histories, and identifying illnesses or injuries. They then create and prescribe treatment plans, which may or may not involve surgery. They also perform surgery and other invasive procedures on a daily basis, working in conjunction with anesthesiologists, surgical nurses, and specialized equipment. Surgeons must always be aware of the potential risks associated with their operations, and they must also monitor their patients’ postoperative recovery and progress.
Most surgeons work in hospitals, although some work for private clinics or surgery centers. They must adhere to the hospital or surgical center’s protocols and regulations regarding patient care, safety, and confidentiality. In addition, they must also maintain a high level of professionalism and ethical standards.
They must attend conferences and meetings, keep up-to-date on new surgical techniques, and participate in medical research as needed. They must also consult with other physicians and medical specialists to determine the best course of action for each patient. In addition to their clinical responsibilities, they must also provide patient and family support and educate them on their treatment options. Surgeons must be able to explain complicated medical terms and concepts in an easy-to-understand manner.
Surgeons must be able to communicate well and remain calm under pressure, as they are frequently faced with life-and-death situations. They must be able to think quickly and decisively in stressful situations, and they must have excellent manual dexterity and attention to detail in order to be successful at their jobs. They must also be able to deal with the emotional stress of operating on seriously injured or sick patients.